Products You May Like
It sounds like a storyline out straight of a science fiction movie: A long, long time ago in a primordial soup far, far away, two separate entities fused in a symbiotic partnership. With their powers combined, an extravagance of living forms flourished, and life as we know it began.
But this far-fetched alien enterprise isn’t a bedtime story. Rather, it’s the science fact that drives the very origins of life on Planet Earth and how you’re able to pedal your bike up that hill.
This is the story of mitochondria, and how tending to these basic building blocks of the body can make you a better athlete—just like building on any strong foundation for training.
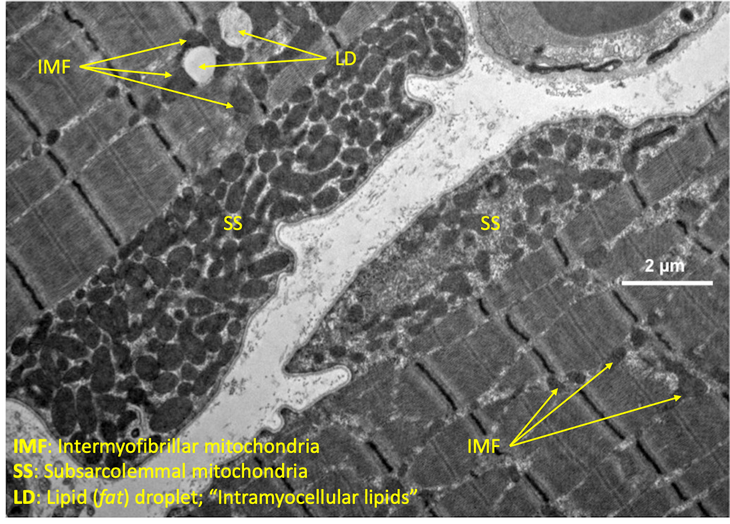
Section divider
What are mitochondria?
If you’re lucky enough to be able to remember back to high school biology class, you may recall being introduced to the mitochondria as the powerhouse of the cell. But there’s more to mitochondria than just keeping the molecular lights on.
“Mitochondria perform numerous functions. One of them is ATP generation,” says Dr. Vamsi Mootha an investigator of the Howard Hughes Medical Institute and a professor of Systems Biology and of Medicine at Harvard Medical School. ATP, or adenosine triphosphate, is the “cellular energy currency, and this is why mitochondria is called the ‘powerhouse of the cell.’”
But these tiny organelles are kind of weird and super fascinating, says Dr. Robert Jacobs, assistant professor of human physiology and nutrition at the University of Colorado, Colorado Springs. “They’re the only component in our cells that also carries its own DNA.”
Mitochondrial DNA is circular like bacterial DNA, as opposed to human DNA, which arranges itself in that recognizable, ladder-like double-helix pattern. Mitochondrial DNA is fundamentally different from what’s found in the rest of the cell, and Jacobs says the theory is that many millennia ago when life on Earth was primarily limited to a single cell or very-few-celled organisms, a bacteria-like substance’s DNA symbiotically merged with our ancestral primordial life form. “The merging of this bacterial mitochondrial life form that was foreign with our ancestral eukaryotic life form allowed life on Earth to actually thrive beyond what it had previously been capable of.”
That’s because mitochondria used oxygen to power itself – a super party trick to be able to pull off in the oxygen-rich atmosphere of Earth’s early days. Previously, oxygen, while plentiful, was a little too volatile for most single-celled organisms to use effectively.
“Oxygen is very combustible,” Jacobs says. “An oxygen molecule is unstable because it wants additional electrons. In the body, that instability will sometimes steal electrons from DNA or our protein and it can be damaging.” (As an aside, this is how free radicals are formed and why every nutritionist tells you to eat plenty of antioxidants because they neutralize free radicals and limit the damage they can cause.)
But before we got to eating lots of leafy green veggies, that initial DNA infiltration set in motion a chain of events. Because this alien substance can handle oxygen and because of mitochondria symbiotically merging with our ancestral, single-celled organisms on Earth, they opened the door to allow evolution to occur.
Today, after billions of years of evolution, “the only reason we can survive with oxygen is because of our mitochondria,” Jacobs says.
These mitochondria power everything you do, from reading a book and singing a song to winning an Olympic-distance triathlon. In short, mitochondria harness the power of oxygen to turn the foods you eat into energy.
Section divider
A variable resource
But mitochondria isn’t all one thing throughout the body. “If you take the mitochondria from two different cell types, for example, the liver versus the heart or skin versus the brain, about half the proteins will be shared between the two of them, but the other half are actually distinct,” Mootha says.
And all those high school biology textbooks that offer an illustration of a cell that showed maybe one or two mitochondria in situ didn’t show the full picture – there can be thousands of mitochondria in a single cell. However, the number of mitochondria varies widely from cell type to cell type. For example, red blood cells have no mitochondria, while heart muscle cells can have more than 5,000 of these tiny structures. The number depends on the cell’s function.
What’s more, because mitochondria constantly fuse and divide, it’s very difficult to count the number of mitochondria at any given time. Therefore, researchers usually count the number of copies of mitochondrial DNA they find in a cell.
Unfertilized egg cells, called oocytes, contain the highest numbers of mitochondria DNA, with upwards of half a million copies. “That’s the Michel Phelps, if you will, of mitochondrial DNA copy number,” Mootha says.
Oocytes are exceedingly large cells, especially when compared to the size of a sperm cell, which carry “very, very few functioning mitochondrial DNA molecules,” says Mootha. Any that manage to find their way into an oocyte during fertilization usually don’t survive. This means that in humans, as a rule, mitochondrial DNA is maternally transmitted.
In muscle cells, the mitochondria don’t take the classic bean shape that appears in most textbooks. Rather, they form networks, or reticulum, which are “elongated, branching structures that kind of run either in parallel to the muscles’ contractile apparatus or in perpendicular to the muscles’ contractile apparatus,” says Dr. Brian Glancy, the Earl Stadtman Investigator at the National Institutes of Health in Bethesda, Maryland.
Jacobs likens these mitochondrial networks to the vast networks of blood vessels and nerves that run throughout the body, just on a nano scale within each cell.
Glancy’s lab is researching how muscle cells build these mitochondrial networks and the different configurations they can take. “The main idea that we’re trying to look at,” Glancy says, “is how do you optimally configure your mitochondria within a muscle cell in order to support muscle contraction or performance?”
It’s all part of a complicated biochemical dance.
“Mitochondria can’t operate by themselves,” says Glancy, adding that mitochondria must pull the components they need to build ATP from fuel sources and then send the ATP onward to other parts of the cell to be used. “So where mitochondria are in relation to all the other parts of the cell has a big impact on how mitochondria work within itself. It’s an integrated system in which mitochondria are operating as a key part.”
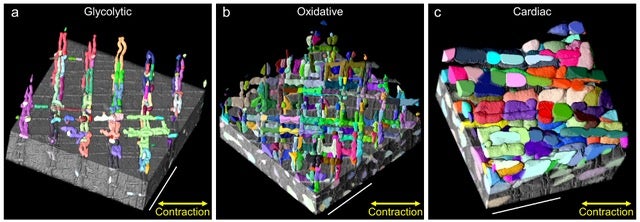
Section divider
Mitochondria and exercise
The number of mitochondria you have in your cells can also vary depending on the stressors you face and other environmental factors.
Dr. David Hood, director of the Muscle Health Research Centre at York University in Toronto, Canada, is an expert in the molecular biology of mitochondria and how they adapt to exercise. He has spent his career studying how mitochondrial numbers within cells increase, a process called biogenesis. He’s also been investigating the lifespan of mitochondria – how they degrade and how new mitochondria replace ones that aren’t working as well. That turnover, or refreshing the pool of mitochondria within the muscle, is aided by exercise.
Hood says his work has shown that the type of training endurance athletes have been doing for years supports good mitochondrial health: “We found that exercise expands the mitochondrial network and muscle to provide more energy.”
A single bout of exercise gets the process going by activating genes to transcribe proteins that will ultimately be translated into proteins that assemble into mitochondria. “Over the course of three or four weeks (of consistent training), you can start to have significant increases in mitochondria. But you have to train with the appropriate intensity, duration, and frequency,” Hood says.
Over the course of three or four weeks (of consistent training), you can start to have significant increases in mitochondria. But you have to train with the appropriate intensity, duration, and frequency.
At the same time, however, “exercise provokes the breakdown and elimination of fragments of mitochondria that are no longer producing sufficient ATP or are producing too many reactive oxygen species (aka free radicals) that are damaging.” This process is called mitophagy, or autophagy of mitochondria, and is a form of cellular recycling that digests damaged organelles and resynthesizes their proteins into fresh new mitochondria. In other words, exercise helps build up your mitochondrial network, but it can also help clean out the less functional organelles that have done their duty.
“Exercise can rejuvenate the mitochondrial pool in an older muscle to help preserve mitochondrial content, preserve energy provision, and help to eliminate those poor-quality mitochondria that exist in muscle,” Hood says.
As quickly as exercise can build up your mitochondria, total rest can bring it down. “If you build up your mitochondria and then stop training, in about one to two weeks, your mitochondrial content will come back down to where it was.”
Because of this, mitochondrial content is described as “labile,” or easily altered. “You’ve got to continue to maintain a level of physical activity to keep the level of mitochondria constant in your muscles,” Hood says.
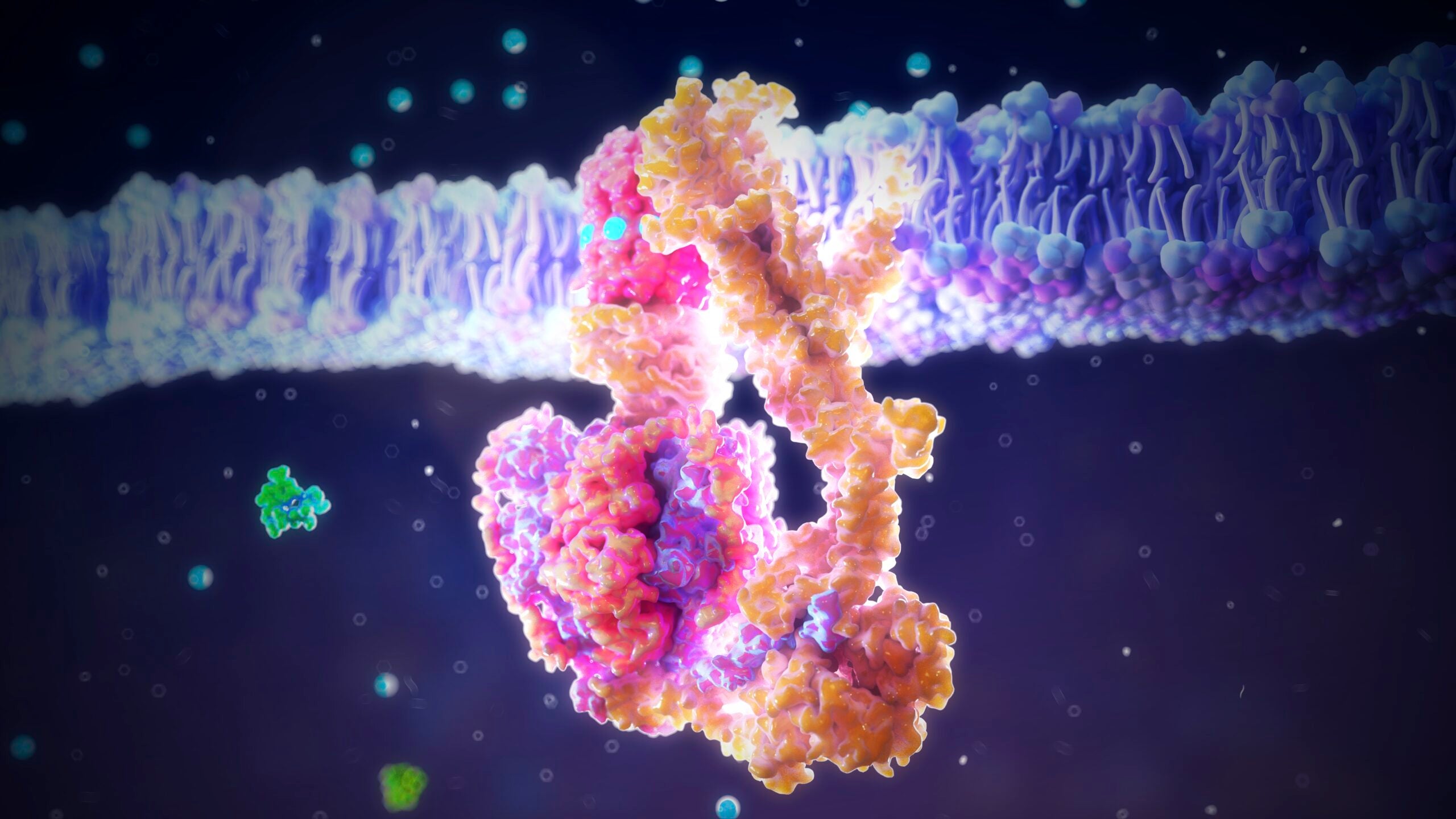
Section divider
The triathlete’s guide to boosting mitochondrial output
If you’re looking for insight as to how best to support your mitochondrial function, “training is the simple answer,” Glancy says.
Mitochondria are highly plastic, meaning they’re receptive to influences like training input. They adapt to help you meet the challenges you’re preparing for.
Hood’s work has helped explain why traditional training plans have long been effective for so many athletes. The concept of training consistently with gradually increasing loads works because it helps your muscles create more mitochondria to power that effort.
In recent years, high intensity interval training (HIIT) has taken off, and there’s good evidence that this is an especially effective training methodology for boosting mitochondrial numbers.
“If you’re a long-distance athlete, most of the time you’re doing long distance-type work,” Hood says. “But frankly, if you want your entire muscle to adapt to training, then you’ve got to inject in your training program some high-intensity exercise intervals. Because if you just go out for a run at 60% of your VO2 max and you do that maybe five days a week, you’re going to recruit about two-thirds of your muscle mass. The remaining one-third of your muscle mass won’t adapt at all, because you’re not using those muscle fibers during your training.”
In other words, while there’s absolutely some value to the long-and-slow distance sessions, you have to pepper in some HIIT workouts within the training plan to develop the whole capacity of each muscle. You’ve got to train both the so-called slow-twitch (endurance) muscle fibers as well as the fast-twitch (sprinting) muscle fibers.
“If you always exercise at the same intensity, those muscle fibers that are being used are certainly going to adapt,” Hood says. But when you need to recruit the rest of the muscle’s power, say to fend off a challenger in a sprint to the finish, you may find yourself coming up short if you haven’t done much sprint training.
Or as Jacobs summarizes it: “Having more mitochondria in our muscles would be analogous to having more cylinders in a gas combustion engine.”
Section divider
Broader implications for endurance runners
While mitochondria spend a lot of their time helping us move, there’s so much more to them besides just producing energy. “Mitochondria are primarily responsible for maintaining cellular energetics but they’re also involved in calcium signaling and they’re involved in the programmed cellular death called apoptosis,” Jacobs says.
And because of their multifaceted role, there are broader implications of the research these scientists are doing. This field is “growing faster than any other aspect of cell biology,” Hood says.
Gaining a better understanding of mitochondria could have enormous implications for understanding the hows and whys of aging and chronic disease.
“Aged muscle has reduced endurance and starts to atrophy,” Hood says. “This leads to the frailty and weakness seen in very elderly people. While aging is a multifactorial process, mitochondria dysfunction contributes to muscle wasting and poor endurance as we age.”
A deeper understanding how mitochondria degrade and are replaced could open up whole new frontiers in medicine. “We’re really trying to understand the entire mitochondria as a whole,” Mootha says. “Some of the strongest signatures of the aging process are declines in mitochondrial number and function.”
So far, we know that mitochondrial health “can be improved with exercise. But I would say the billion dollar question in the aging field right now with respect to mitochondria is ‘cause or consequence,’” Mootha says.
If scientists can unravel whether chronic diseases cause mitochondrial quality and quantity to decline, or whether a decline in mitochondria causes chronic disease, that could lead to novel therapies for a wide range of diseases from diabetes and heart disease to cancer and kidney failure.
In pursuit of this better understanding, Glancy hopes to learn how to gain control over the structure of mitochondrial networks, in terms of making them different sizes or shapes or where they can sit in the cell.
“We’re trying to learn how to do that while also trying to understand what the impact is doing—if we change anything in a cell, something is going to get better but something is gonna get worse. So we’re trying to understand the consequences of changing structure and changing the different configurations of the mitochondria network,” Glancy says.
Learning what’s optimal might provide key insight for solving a range of rare mitochondrial diseases. “By understanding the normal functions and the tradeoffs of putting more or less mitochondria and putting them in different places in the cell, that has an impact on lots of different diseases,” Glancy says. “If we understand what’s normal, then when something goes wrong, we’re better equipped to figure out how to fix things.”
Jacobs predicts that in perhaps the next decade, as our understanding of these mighty mitochondria deepens, you might get your mitochondrial health checked when you visit the doctor, just like they check your blood pressure.
One noninvasive measurement technique uses an oversized blood pressure cuff that wraps around the thigh. Through a series of inflations and deflations, the device can determine mitochondrial function in the muscles. While this technique isn’t available in clinical settings currently, “it very much could and should be,” Jacobs says. “Finding out how your mitochondria are working using that technique could be so much more valuable in relation to your overall risk of all-cause mortality than a blood pressure measure.”
Because mitochondria are powerhouses of not only the cell, but your overall health and well-being. “As goes our mitochondrial health, so goes our whole body health,” Jacobs says.